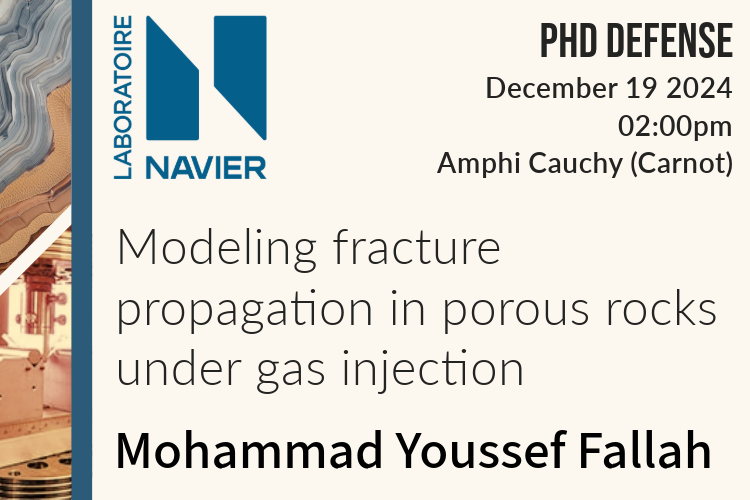
PhD Defense – Mohammad Youssef Fallah
- Post by: Mathias Lebihain
- 16 December 2024
- No Comment
Mohammad Youssef Fallah, a PhD candidate in the Geotechnics and Multiscale teams, will defend his dissertation titled “Modeling fracture propagation in porous rocks under gas injection" on Thursday, December 19, at 2:00 PM, in the Cauchy amphitheater.
Composition of the jury:
-
Laura Blanco-Martín – Mines ParisTech (Reviewer)
-
Pooneh Maghoul – Polytechnique Montréal (Reviewer)
-
Christian La Borderie – Université de Pau et des Pays de l’Adour (Examinator)
-
Jean-Michel Pereira – École des Ponts (Examinator)
-
Amade Pouya – École des Ponts, Université Gustave Eiffel (Thesis Director)
-
Laurent Brochard – École des Ponts (Co-Supervisor)
-
Minh-ngoc Vu – ANDRA (Co-Supervisor)
-
Christophe De Lesquen – ANDRA (Co-Supervisor)
-
Anne-Catherine Dieudonné – TU Delft (Guest)
-
Séverine Levasseur – ONDRAF/NIRAS (Guest)
You can follow the defense online on Microsoft Teams.
Abstract
Show the abstract
As part of ANDRA’s Cigéo project for the underground disposal of radioactive waste in Callovo-Oxfordian clays, a network of fractures is generated around the galleries during excavation. This fracturing has a typical mixed-mode I/II propagation profile, resulting from the instantaneous discharge of the clays. The topology and spatial extent of the induced fracture network have been extensively studied by ANDRA around the structures of the Laboratoire Souterrain of Meuse/Haute-Marne (LSMHM). Over the long term, this fracture network will also be subjected to additional stress from gases (H2), mainly resulting from corrosion of the metallic components of the repository (reinforcement of the galleries and MA-VL cells, lining of the cells and HA packages, etc.) and from radiolysis of the water. The accumulation of these gases leads to pressurization, which can affect the fractured zone. ANDRA is conducting studies into the risks associated with gas pressurization. In particular, gas fracturing tests have been carried out in boreholes drilled in the LSMHM. Analysis of these tests requires a model capable of predicting the response of a fractured porous medium under gas stress, which in particular involves coupling gas migration in an unsaturated medium and the poro-mechanical response of the medium with crack propagation. The aim of this thesis project is to study the conditions of this gas fracturing through theoretical physico-mechanical analyses and numerical simulation, taking into account unsaturated hydromechanical (HM) couplings with rock and fractures. This work is based on data supplied by ANDRA and on existing numerical tools, in particular the Disroc code, in which the coupling models required for this analysis have been defined.
We have developed a two-phase model that takes into account the compressibility of the gas phase. We recalled the main concepts of unsaturated fracture modeling in a porous medium, and considered all the mechanical and transport mechanisms involved. The rock mass around the gallery is a porous medium under unsaturated conditions, i.e., filled with a mixture of liquid (water) and gas (air). Hydrogen flow can occur by diffusion in the gas phase or by dissolution and advection in the liquid phase. In this work, we focus on the behavior of the porous medium both mechanically and hydraulically. We began with a literature review focusing on the main gas migration phenomena identified in argillite. We then formulated the two-phase, liquid-gas, unsaturated problem for the porous matrix, the liquid in our case being water and the gas hydrogen. To do this, we introduced the two-phase flow equations from the conservation equations for water and hydrogen. By integrating Fick’s and Darcy’s laws, we were able to establish the complete system of two-phase flow equations. The same process was applied to fractures: we established the unsaturated two-phase flow equations in fractures by writing the conservation equations for water and hydrogen and for flow as a function of the quantities adapted to fractures. This study employs the cohesive zone model to model fracture propagation. The results have been validated by comparison with semi-analytical solutions for simple cases, confirming their consistency with the principles of fracture mechanics.
We carried out an initial sensitivity analysis to determine the most important parameters controlling flow, as well as the order of magnitude of the contribution of each phenomenon (diffusion, advection) to overall hydrogen transport in different regimes. Our numerical simulations consider a fractured porous medium with the possibility of flow both in the fractures and in the porous matrix, and with mass exchange between them. We validated the equation implementations in our 2D simulations with the Disroc finite element code by running two benchmarks with the FEniCS code for two-phase flows in the rock matrix and in the fracture.
As part of ANDRA’s Cigéo project, this study examines how existing fractures around a gallery, created by excavation, can be reactivated and propagated under the effect of an increase in gas pressure. These simulations take into account the in-situ stresses and elastic anisotropy of the materials for different fracture geometries around the gallery, which are representative of the excavation-induced fractured zone for two orientations, parallel and perpendicular to the major horizontal stress. This study focuses on the examination of scenarios relevant to the long-term disposal of radioactive waste. During gas pressurization processes, we focus mainly on the study of multiphase flow phenomena and their coupling with rock matrix mechanics and in particular with fracture reactivation. We have modeled the progressive diffusion of gas in the geological formation and determined the critical conditions for fracture propagation around the gallery. We also analyzed the impact of various gas pressure evolution scenarios on fracture propagation rates. According to the results, under the conditions of gas production and pressure evolution predicted by ANDRA, no fracture reactivation is observed. We found that under higher loadings, the fracture propagation occurs when the loading pressure exceeds the in-situ stress orthogonal to the fracture, and the rate of fracture propagation increases with the imposed gas pressure. The critical pressure for propagation remains well above the maximum pressure currently estimated in long-term storage (7-8 MPa). We also analyzed the effect of stress and material anisotropy on fracture propagation direction and length. Analysis of these complex physical mechanisms is imperative to guarantee the containment capacity and long-term safety of the repository. This research has also led to a better understanding of the contributions of poro-mechanical couplings to fracture propagation on different time scales.