Researchers at RMP investigate transport phenomena (liquid, fluid, humidity, heat, acoustic waves) and how these phenomena are affected by the geometrical constraints present in porous materials, e.g. fibre stacks, solid foams with open / closed porosity and bio-based materials …
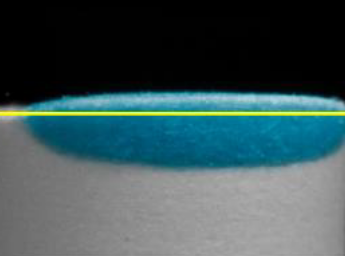
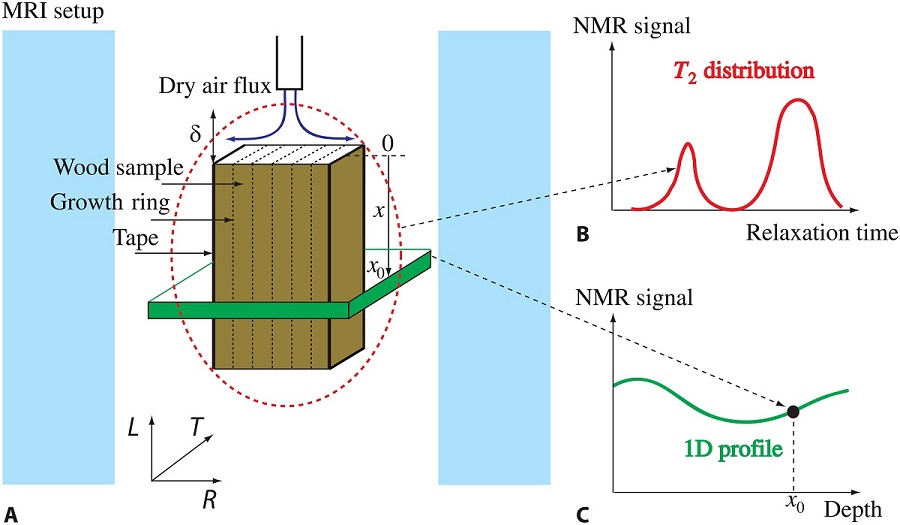
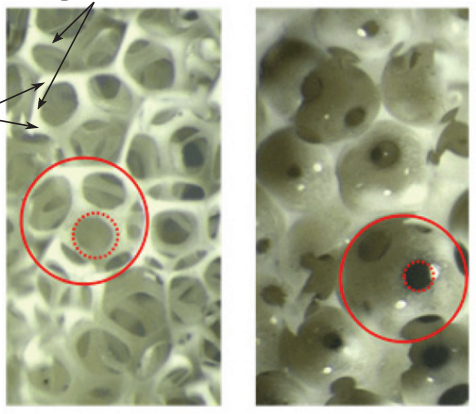
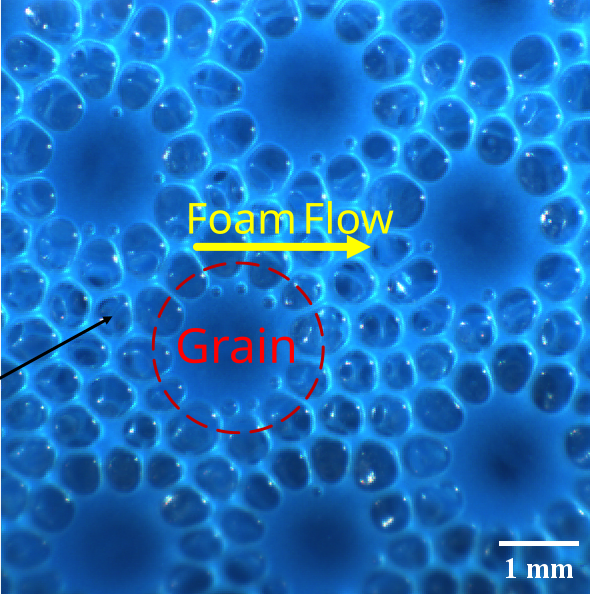
Hygrothermal properties of bio-based materials
Philippe Coussot, R. Sidi-Boulenouar, B. Maillet, Kang Hu (Postdoc), Yuliang Zou (Postdoc), Nicolas Daunais (PhD), Van-Truong Nguyen (PhD), Luoyi Yan (PhD), Karen Mourda (PhD),
Collaborations : S. Caré (MSA), L. Brochard (ME)
How does water enter wood and induce swelling ? Why are we more comfortable wearing cotton, flax linen or hemp clothes ? Why does it take so long to dry wood or paper ? Why is it healthier to live in a house made of bio-based materials ? At the heart of these questions, we find the remarkable capacity of these materials to absorb liquid water or water vapour from the environment through hydrogen bonds, which create water nano-sized water inclusions between the cellulose microfibrils. This “bound" water can make up to 30% of the dry mass of the material. It evaporates under a dry ambient atmosphere, hence regulating the ambient humidity. Bound water also induces the swelling or the retractation of their matrices proportionally to the amount of bound water. Bound water can also diffuse strikingly fast through the cellulose fibres. Bound water transport and its exchange with the liquid and vapour (standard) lead to complex and original transport properties in cellulose-based or plant-fibre-based materials.
For instance, spontaneous water imbibition in wood is not governed by capillary effects but rather by diffusion of bound water ahead of the liquid front, which slows down the imbibition dynamics by several orders of magnitude. Conversely, wood drying is controlled by bound water diffusion which extracts the free water stored deep inside the sample, leading to a slow, two-step diffusion process. We observe and quantify these phenomena with specific scientific tools, among which NMR and MRI are particularly efficient as they access very low water content in solids and allows us to separate the signals from bound and free water. We have determined the transport and diffusion coefficient of bound water in wood samples, in a fibre network (bound water jumping from one fibre to another when they are in contact) and even along a single cellulose fibre.
These studies will carry on in the framework of the PHYSBIOMAT ERC Advanced Grant, which aims to predict the hygrothermal behabiour of bio-based construction materials. We will characterise the elementary transport phenomena on model and real materials, along with a detailed modelling of the coupling between heat and humidity transfers. The first articles published on this topic underline how one must perform sorption dynamic measurements in a controlled fashio. They also show how it is possible to separately determine the diffusion coefficients of water vapour and bound water in a cellulose fibre structure; and to then deduce a global transport model from these coefficients, taking into account exchanges between the two phases.
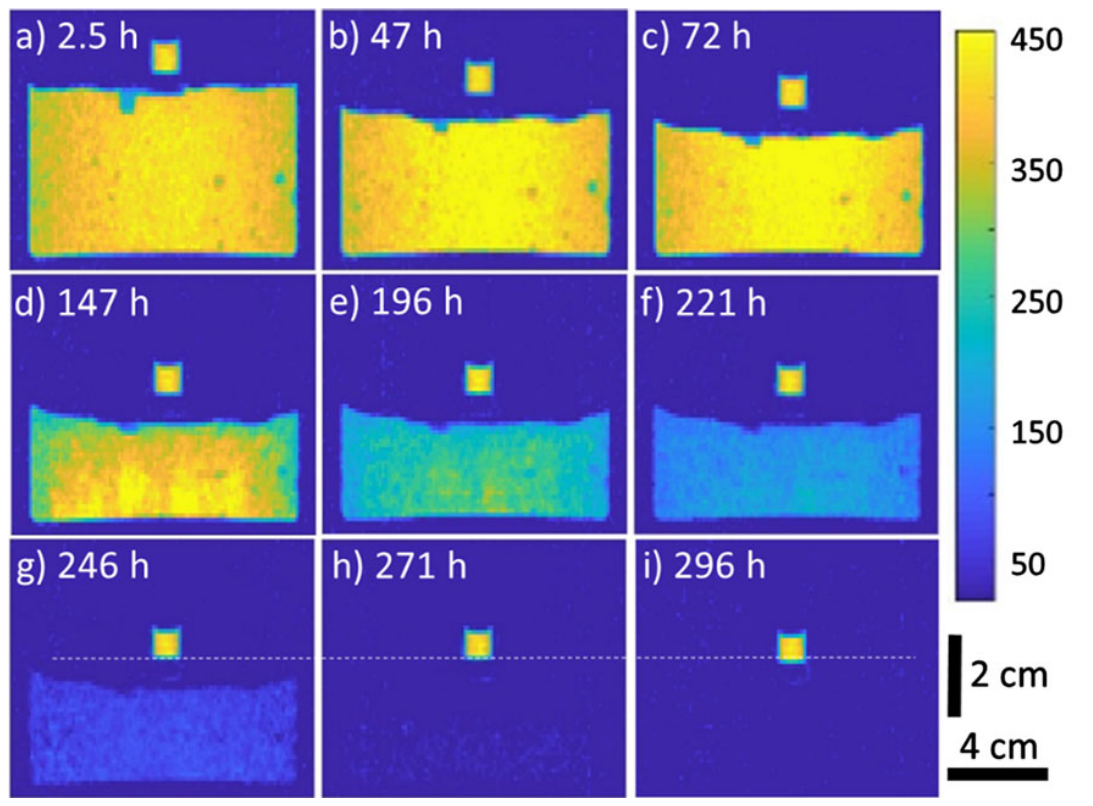
Dynamical drying of a cellulose slurry under an air flux. Water content is measured by MRI. From Ben Abdelouahab, Cellulose 28, 28, 5321–5334, (2021)
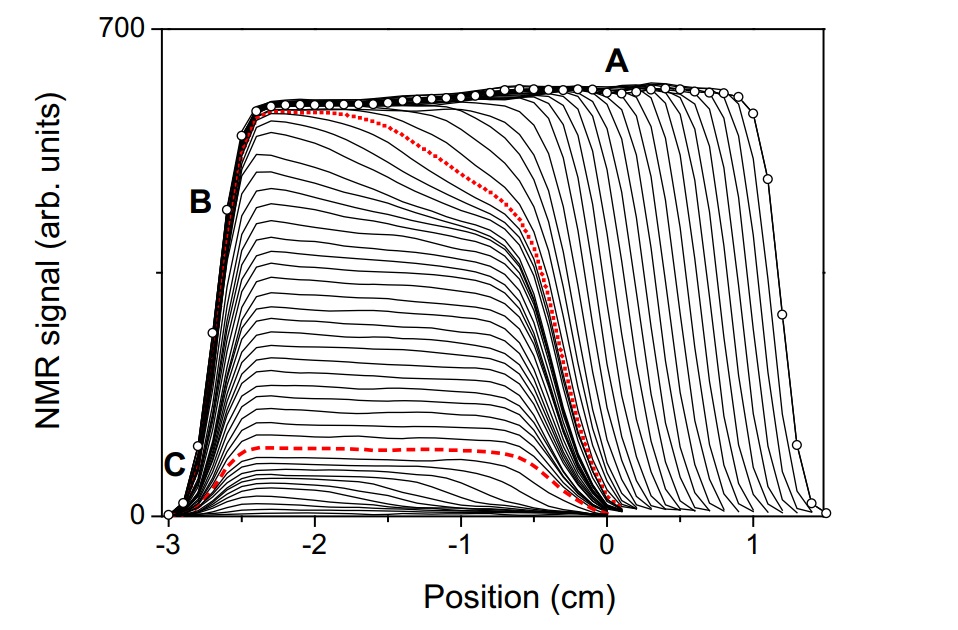
One-dimensional profiles of a cellulose slurry drying under a (vertical) air flux. We notice that drying is a two-step process. From Ben Abdelouahab, Cellulose 28, 28, 5321–5334, (2021)
References
- Understanding mechanisms of drying of a cellulose slurry by magnetic resonance imaging, N. Ben Abdelouahab, A. Gossard, X. Ma, H. Dialla, B. Maillet, S. Rodts and P. Coussot, Cellulose 28, 5321–5334 (2021)
- How Bound Water Regulates Wood Drying, H. Penvern, M. Zhou, B. Maillet, D. Courtier-Murias, M. Scheel, J. Perrin, T. Weitkamp, S. Bardet, S. Caré, and P. Coussot, Phys. Rev. Applied 14, 054051 (2020) | ❦ Editor’s Suggestion & Featured in Physics | Featured on the CNRS website
- Critical Role of Boundary Conditions in Sorption Kinetics Measurements, Y. Zou, B.Maillet, R. Sidi-Boulenouar, L. Brochard, and P. Coussot, Langmuir 39, 51 (2023)
- Unveiling moisture transport mechanisms in cellulosic materials: Vapor vs. bound water
Y. Zou, B. Maillet, L. Brochard and P. Coussot, PNAS Nexus 3, 450 (2023)
NMR Relaxometry for Porous Materials
R. Sidi-Boulenouar, B. Maillet, P. Coussot
Porous materials are largely present in our daily lives: soil, wood, bricks, concrete, sponges, clothes and fabrics … It is then crucial to identify how liquid — which may also transport particles and ions — enters the porous media or can be extracted from them. High-resolution X-ray microtomography, neutron imaging or Magnetic Resonance Imagnig (MRI) usually do not provide quantitative measurements at the micro- and nano-pore size.
We have developed an original NMR-based technique which provides direct, dynamic, quantitative information on the liquid distribution inside porous materials; we can then track the liquid fraction variations with time associated to transport of fluids or phase changes. This dynamic NMR relaxometry relies on successive measurements of the probability density of the NMR relaxation times of the liquid inside the material, obtained from a Laplace transform the of NMR signal during its relaxation. We analyse the time evolution of this probability density, notably the peak location and width of the NMR signal, or the local area under the curves representing the fraction of fluid associated to every peak. We have then measured and described in detail the physical mechanisms (spatial heterogenity, adsorbed molecular layers, wetting and dewetting, bound/free water transport, etc.) governing water imbibition and drying in nano-porous materials, cellulose, bi-porous materials or in wood samples.
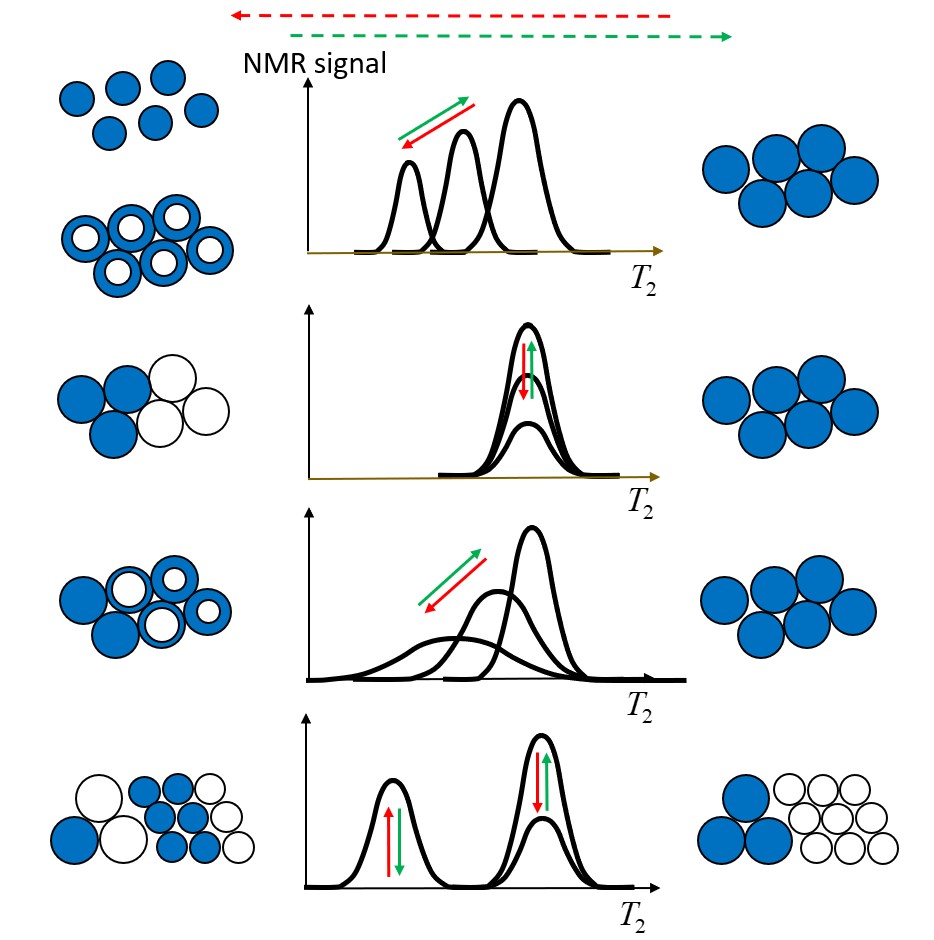
NMR Relaxometry : the characteristic relaxation time T2 of water decreases as water confinement increases (here, represented by a change from water in a large sphere to water in a smaller sphere, or in a ring). The overall distribution of the relaxation times T2 obtained by NMR then measures the amount of water present at each ‘degree’ of confinement in a given material.
References
- “Dynamic NMR relaxometry” as a simple tool for measuring liquid transfers and characterizing surface and structure evolution in porous media, B. Maillet, R. Sidi-Boulenouar, P. Coussot, Langmuir 38, 15009-15025 (2022) | Invited Featured Article | Editor’s choice
- Two-step diffusion in cellular hygroscopic (plantlike) materials, M. Cocusse, M. Rosales, B. Maillet, R. Sidi-Boulenouar, E. Julien, S. Caré, P. Coussot, , Science Advances, 19, eabm7830 (2022)
Acoustic Permeability of Solid Foams
O. Pitois, V. Langlois, Y. Khidas
We study how foam morphology — their pore size distribution, the fraction of open membranes and the size distribution of the membrane openings — affects their acoustic properties and their performance in applications in the construction industry (acoustic isolation, reverberation control). Our aim is to bring a comprehensive view of the relation between the foam microstructure and its acoustical properties. The fraction of open membranes and the opening size distribution is measured by X-ray microtomography. Acoustical measurements are conducted in impedance tubes (Kundt tubes) with three microphones. We conduct indirect numerical simulations to capture the acoustic properties of these media. These simulations are based on semi-phenomenological models decoupling the various physical phenomena — viscous dissipation, heat conduction, mechanical vibrations — during the wave propagation in porous media. This Johnson-Champoux-Allard-Lafarge (JCAL) model considers a rigid solid matrix and links the acoustical properties of the overall medium with its viscous and thermal properties, i.e. viscous permeability, tortuosity, characteristic viscous length, thermal permeability and thermal characteristic length.
Our work consists in:
- Compute all these parameters through numerical approaches : finite elements, or a pore-network model for the viscous permeability and the tortuosity applied to different microstructures
- Design physical models describing these viscous and thermal properties.
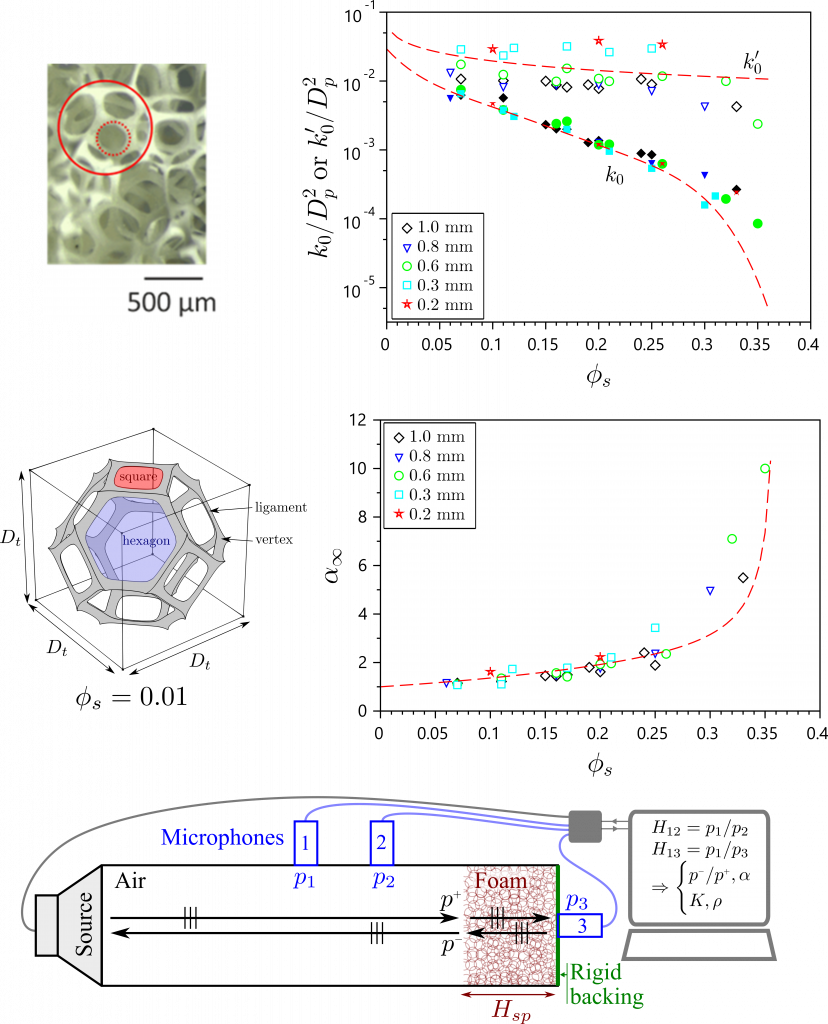
Normalised Darcy permeability k0 and tortuosity α∞ in solid foams with increasing solid volume fraction ϕs.
References
- Polydisperse solid foams: Multiscale modeling and simulations of elasto-acoustic properties including thin membrane effects, C.T. Nguyen, V. Langlois, J. Guilleminot, F. Detrez, A. Duval, M. Bornert, P. Aimedieu and C. Perrot, Int. J. Solids Struct. (2022).
- Acoustics of monodisperse open-cell foam: An experimental and numerical parametric study, V. Langlois, A. Kaddami, O. Pitois, C. Perrot, JASA (2020).
- Tuning membrane content of sound absorbing cellular foams: Fabrication, experimental evidence and multiscale numerical simulations, V.H. Trinh, V. Langlois, J. Guilleminot, C. Perrot, Y. Khidas, and O. Pitois, Mater. Des. 162, 345 (2019).
- Electrical conductivity and tortuosity of solid foam: Effect of pore connections, V. Langlois, V.H. Trinh, and C. Perrot, Phys. Rev. E 100, 013115 (2019).
Liquid Foams in Porous Media
O. Pitois, V. Langlois, Y. Khidas
Foam injection in porous media has been largely studied by the Oil & Gas industry, and more recently to develop environmental remediation processes to remove contaminants from soils. The complex rheology of liquid foams allows it to invade the porous medium in a homogeneous fashion, more so than simple liquids. This is particularly the case if the permeability of the medium is high. For environmental remediation applications, we need to know how fast the foam liquid flows in the ensemble formed by the porous medium filled with liquid foam.
We have investigated this process using a model experiment where the pore size, the bubble size, the liquid volume fraction and the interface behaviour (frozen or mobile depending on the choice of surfactant) are controlled. The liquid permeability of the samples, normalised by the square of the bubble size, decreases over several orders of magnitude when r — the ratio between the bubble and the grain size — increases. The relative permeability, i.e. that of the foam-filled porous media divided by the permeability of the same porous material filled with liquid, shows an optimal value as a function of r for frozen interfaces. In contrast, it increases monotonically with r for mobile interfaces.
The permeability of porous materials filled with foams of mobile and frozen interface then show two regimes as a function of r. For r ≲ 0,25, the ratio is equal to that measured for un-confined foams, whereas for higher values of r, the permeability ratio increases by one order of magnitude. This result seems to be related to the microstructural change that we notice when r reaches 0.5. In that case, a liquid network different from the unconfined foam activates: it is composed of surface canals and liquid bridges, the former linking the latter even for very low liquid volume fractions. We have modelled these changes, with the main result being that the foam inside the pores is drier compared to a non-confined foam as the liquid is being drawn by the surface canals and the liquid bridges. Having drier foams explains the sharp decrease of the liquid permeability of the foam. Besides the liquid drainage, we expect all foam properties to be impacted by the confinement.
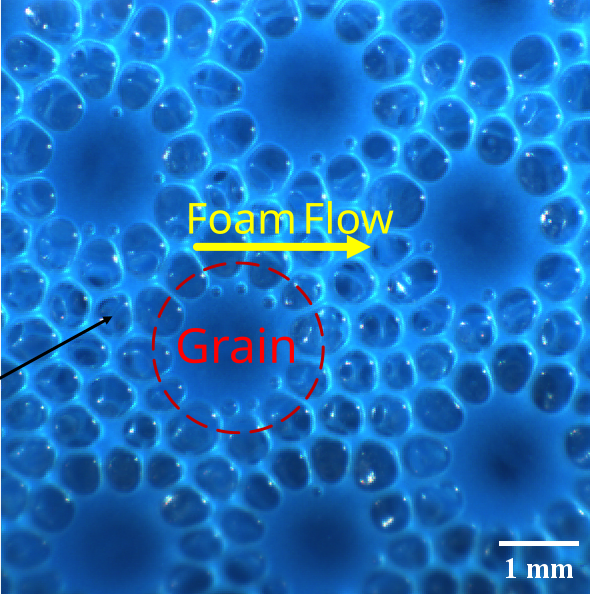
References
- Liquid relative permeability through foam-filled porous media: Experiments, M. Ceccaldi, V. Langlois, M. Guéguen, D. Grande, S. Vincent-Bonnieu and O. Pitois , Phys. Rev. Fluids 8, 024302 (2023)
- Daisy-shaped liquid bridges in foam-filled granular packings, Pitois O., Salamé A., Khidas Y., Ceccaldi M., Langlois V. and Vincent-Bonnieu S., Journal of Colloid and Interface Science 638, 552-560 (2023)